Summary
Losses in physical channels, such as optical fibres, limit existing quantum communication systems to modest distance ranges. Since amplification of quantum signals is fundamentally not possible, we look to extend the range and functionality of these quantum channels by adding quantum memory nodes that can daisy-chain multiple lengths of quantum channels through entanglement and thus extend the communication distance — an approach known as ‘quantum repeater’. Quantum repeaters are by necessity hybrid devices, as they connect flying qubits (photons) to small processors for error correction and privacy amplification. In this project we develop a two-node proof-of-principle hybrid quantum repeater system. We generate entangled photon pairs from quantum dots embedded in semiconductor nanowire and store them in atomic quantum memories following a frequency up-conversion. We expect this will enable quantum key distribution over long distances at rates exceeding those possible through a direct link. The photon-pair sources, the frequency converters, as well as the quantum memories will be implemented in compact on-chip platforms. This novel approach combines the advantages available from a deterministic and tunable solid-state source of bright entangled photon pairs with the potential for high-efficiency long-lived quantum memory that is achievable with laser cooled atoms. The ultimate goal is to achieve a working pair of quantum repeater nodes at practically relevant wavelengths that would lead to useful rates for long-distance quantum key distribution.
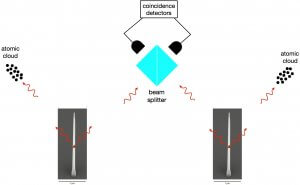
Figure 1. The two quantum dots (red triangles embedded in semiconductor nanowires) produce pairs of entangled photons. One photon from each pair is stored in an atomic ensemble memory, while the other photon is sent into a coincidence measurement setup, which generates entanglement between the two atomic ensembles.
Related Content
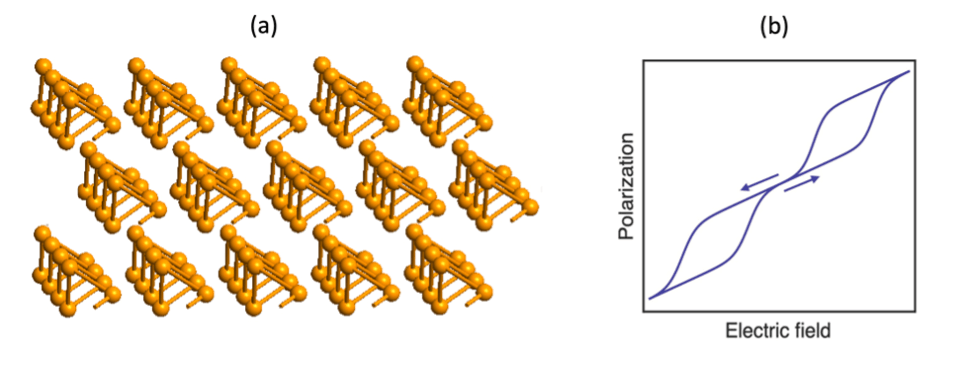
Enabling Next-Generation Sustainable Computing through Novel Multi-Valued-Logic Quantum Devices
As the demand for digital services grows, so does the need for data centres and transmission networks. Unfortunately, these data systems consume vast amounts of energy, resulting in nearly 1% of all energy-related greenhouse gas emissions. This project aims to invent novel quantum devices for highly energy-efficient computing that may help reduce the global digital […]
June 12, 2023
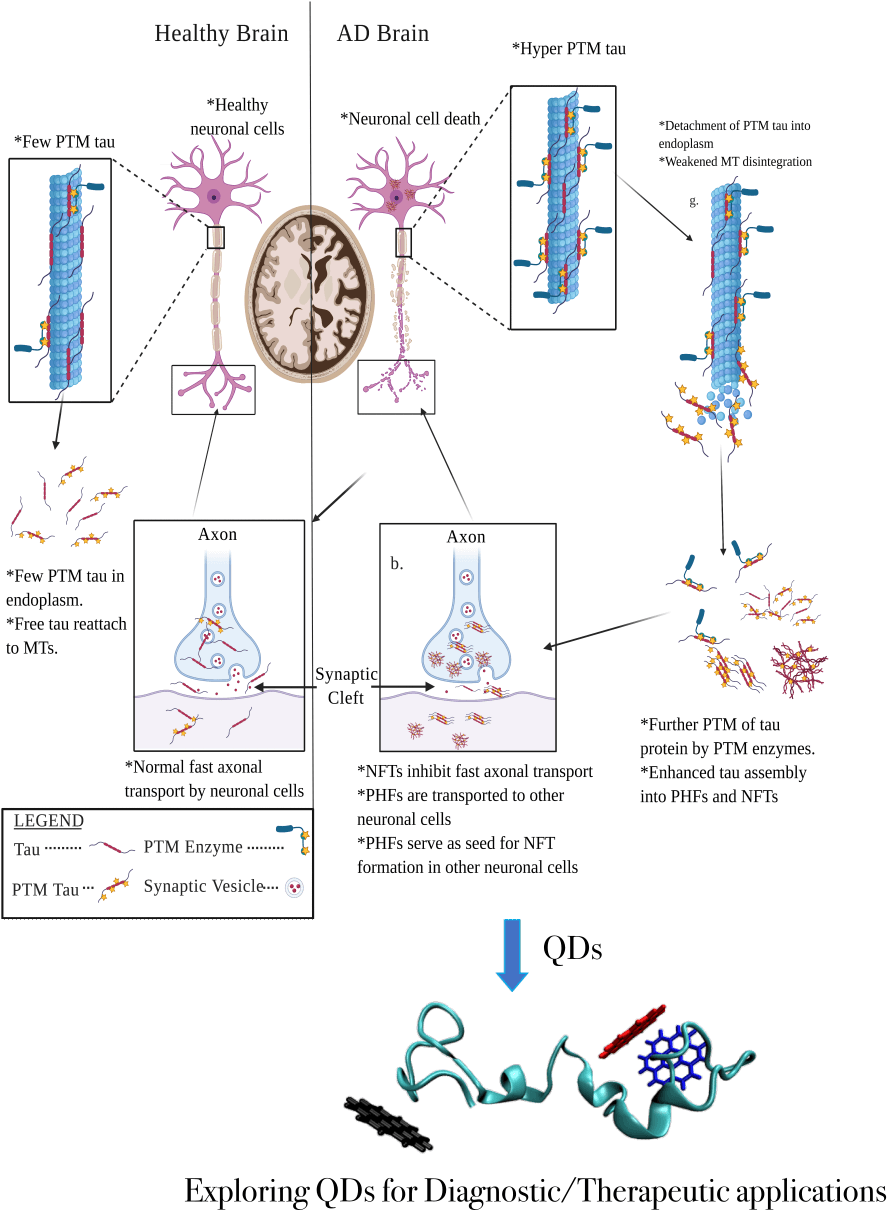
Identifying the Potential of Quantum Dots to Detect and Disrupt Tau Protein Aggregation in Alzheimer’s Disease
Specific tests for Alzheimer’s disease (AD) diagnosis are currently unavailable, despite AD being the leading cause of dementia. One hallmark of AD progression is the aggregation of tau proteins into paired helical filaments and neurofibrillary tangles, which is accelerated by the hyperphosphorylation of Tau proteins. However, the mechanism by which the hyperphosphorylated tau accelerates protein […]
March 27, 2023
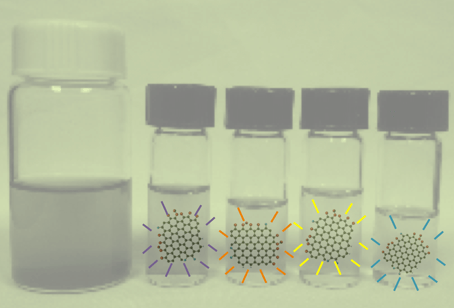
Zero-Dimensional Quantum Materials for the Next Generation of Highly-Selective Chemical Sensors
Summary Heavy metals are a major public health concern and their on-site detection in water supplies is not well served by existing lab techniques. We develop a new multi-modal platform comprising functionalized quantum dots of two-dimensional materials (2D-QDs) for the sensing of four highly-toxic heavy metal pollutants (arsenic, cadmium, lead and mercury). The zero-dimensional […]
March 11, 2019
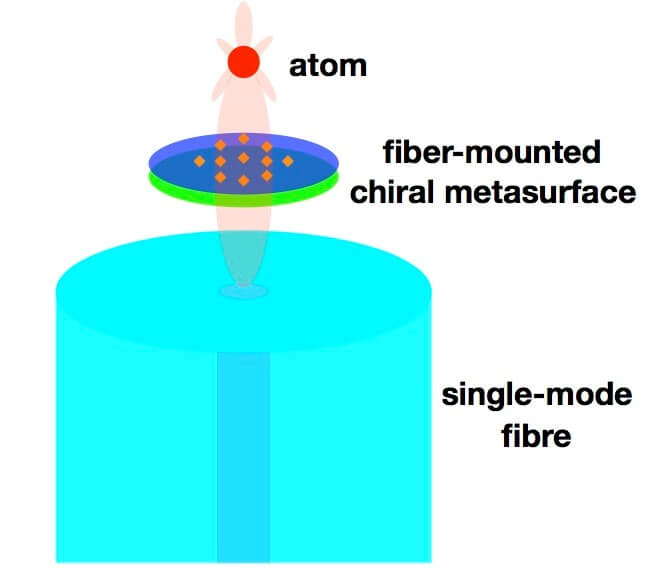
Chiral Quantum Antenna Based on Multilayer Metasurface
Summary Individual atoms can act as stationary qubits and thus serve as nodes in quantum computing networks or as memories for quantum repeaters. However, to successfully use qubits based on single atoms suspended in free space, photons emitted by a single atom need to be efficiently collected. Conventionally, this can be done with high […]
September 20, 2018