Summary
Arrays of orbital angular momentum (OAM) states of light are a new form of structured light so far relatively unexplored in quantum information science. Unlike spin angular momentum of light, which is related to light’s polarization and covers two dimensions, OAM states, sometimes described as ‘donut beams’ due to the shape of the field intensity distribution in their cross section, are in principle an infinite dimensional system and can be used to carry much more information per photon. In this project, we generate arrays of entangled orbital angular momentum beams and explore the utility of the spatially entangled photons in quantum communication protocols, such as remote state preparation. In collaboration with Dmitry Pushin, David Cory, and Thomas Jennewein, we study the propagation of entangled OAM arrays and their self-imaging capabilities known as the Talbot Effect, which hold promise for developing a new method to measure OAM. As we learn to control the spatial patterns of these light beams we expect they may find application in sensing of periodic optical structures in materials.
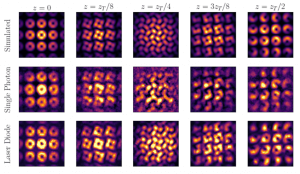
Figure 1. Simulated and observed intensity distribution in orbital angular momentum arrays as they propagate over different fractional Talbot lengths
Related Content
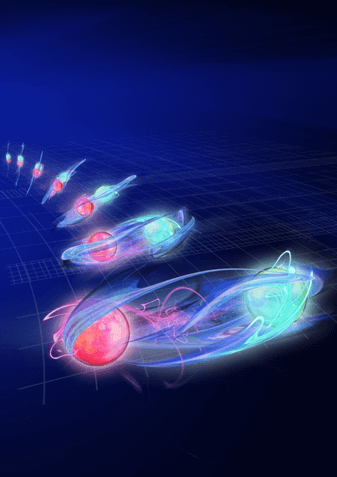
Quantum Simulations of Fundamental Interactions
Summary To address questions in modern physics such as “what is the structure of matter inside neutron stars?” we need better computational methods to evaluate the interplay of fundamental forces between elementary particles. To-date the response to such questions rests on numerical computer simulations that are inherently limited. In this project, we develop new theoretical […]
April 18, 2019
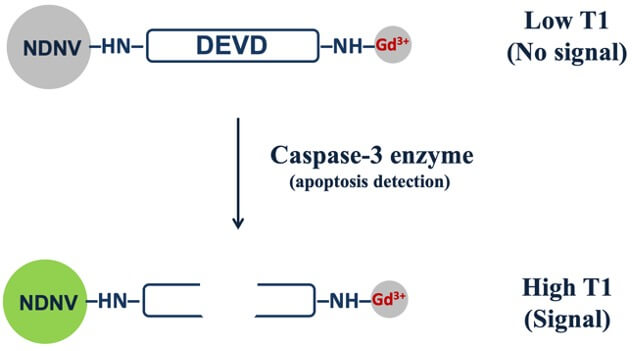
Functionalized Nanodiamonds for Sensing Biochemical Processes
Summary Chemotherapy is limited by the failure to clinically monitor the efficacy of the treatment in real-time, which results in suboptimal chemotherapy being given for a prolonged period. Predicting the outcome of chemotherapy immediately after drug administration can increase diagnostic accuracy, efficacy outcomes, and successful treatment. Quantum nanodiamond sensors can be used as optical sensors […]
August 31, 2022
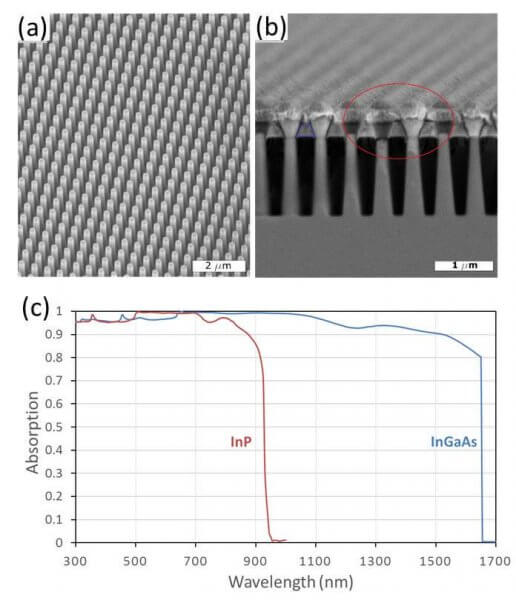
Next Generation Quantum Sensors
We are developing new semiconductor p-n junctions and designing novel nanowire arrays that have the potential to significantly enhance the ability to detect light at the single photon level over an unprecedented wavelength range from the ultraviolet to infrared.
June 1, 2017
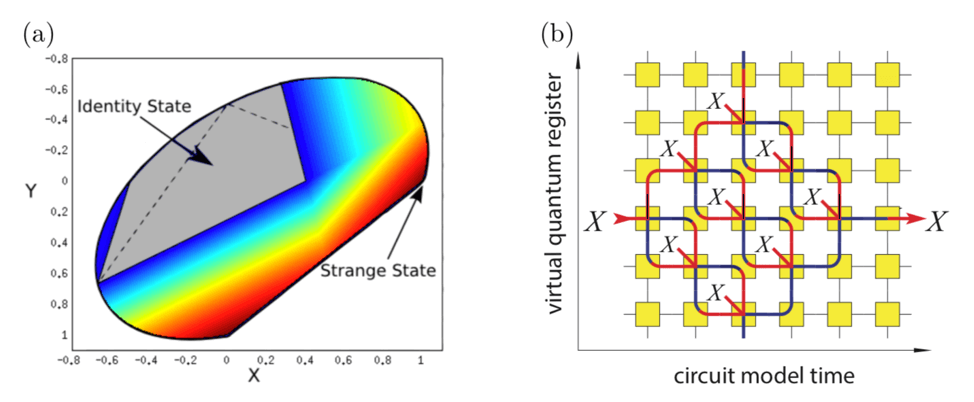
Quantum Computational Resources in the Presence of Symmetry
Summary Fault-tolerance is essential to the performance of quantum technologies, but known schemes are extremely resource intensive. Thus, improving existing schemes or inventing new schemes is of central importance. This joint project is based on the realization that fault-tolerance schemes make use of symmetries in fundamental ways, and that studying the problem of fault tolerance […]
March 13, 2019