Summary
Scaling solid-state quantum processors to a useful threshold while maintaining the requisite precision in quantum control remains a challenge. We propose a quantum metal-oxide-semiconductor (QMOS) architecture operating at cryogenic temperatures that is based on a network/node approach as a means to scalability. By working with QMOS, we benefit from the deep investments and advances that have been made in conventional CMOS device processing, and natural compatibility with CMOS integration. The architecture uses one of the most promising error correction schemes: topological stabilizer codes acting on a two-dimensional qubit arrays, also known as surface codes. The network/node approach is advantageous because it separates the surface code operation into two fundamental parts: local node operations involving a handful of qubits, which should be feasible to demonstrate in the near-term, and medium range entanglement distribution based on electron shuttling, which is challenging but can be developed in parallel. A major focus of this project is to simplify QMOS devices – reducing the number of gate electrodes per device, even down to a single electrode. The team led by Dr. Baugh with collaborators Dr. Lan Wei and Dr. Michel Pioro-Ladrière combines expertise in electrical engineering and CMOS integrated design, QMOS fabrication and physics. By testing the viability of a network/node approach, this project charts a path toward a large-scale quantum information processor in silicon.
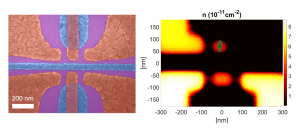
Figure 1. (left) A false-colour scanning electron microscope image of two metal-oxide-semiconductor (MOS) quantum dots, where one is placed as the mirror image of the other. Purple represents SiO2/Si, blue represents the aluminum screening gates, and red represents the aluminum accumulation gates. In this simplified design, the tunnel coupling between the dot and the source/drain reservoirs is controlled by the physical gap between metal gates and the voltage applied to the source/drain accumulation gates. (right) Nextnano simulation of the charge density in the silicon two-dimension electron gas with top gate voltages typical of device operation. Here, the lower device is used as a charge sensor (single electron transistor) to readout the charge state of the upper dot, which can be tuned to single electron occupancy to host an electron spin qubit.
Related Content
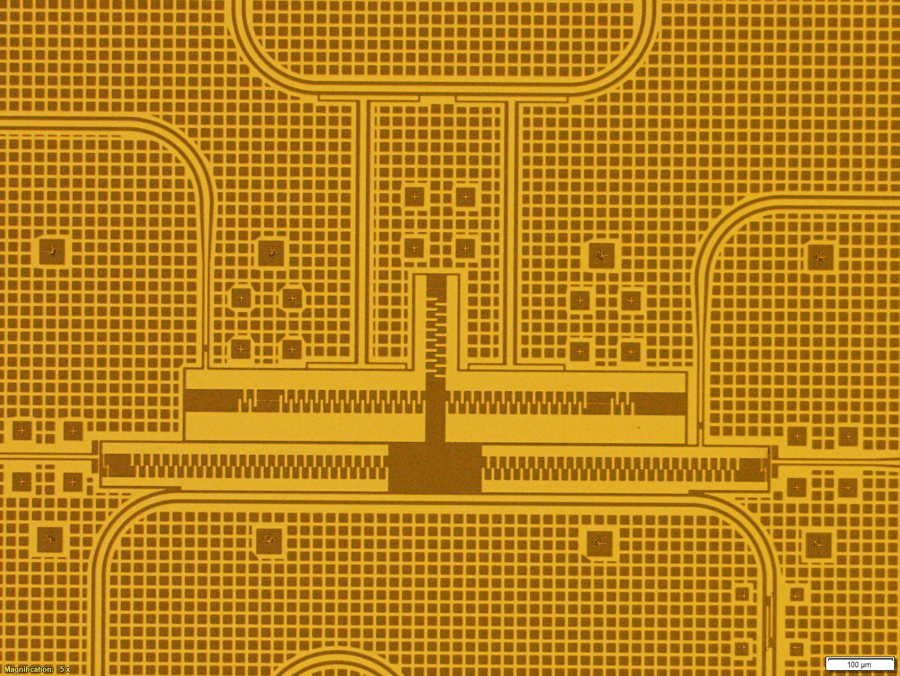
Quantum Simulation of Strongly Coupled Field Theories
Strongly-coupled field theories describe both fundamental and applied quantum problems.
August 10, 2017
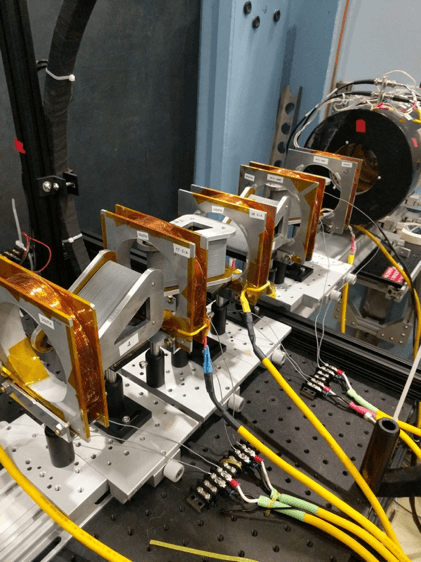
Applications of Neutron Interferometry and Structured Neutron Beams
Summary Neutrons are a powerful probe of matter and physics due to their Angstrom size wavelengths, electric neutrality and relatively large mass. In this project, we develop quantum sensors that exploit these attributes to increases the precision of measurements of fundamental forces and materials structure. With David Cory, Alexander Cronin of the University of Arizona, […]
July 31, 2018
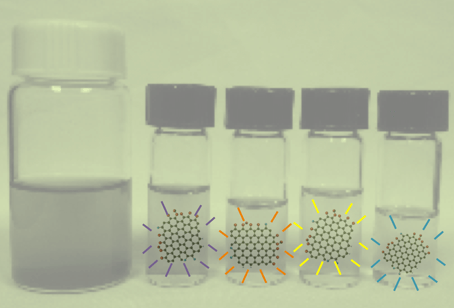
Zero-Dimensional Quantum Materials for the Next Generation of Highly-Selective Chemical Sensors
Summary Heavy metals are a major public health concern and their on-site detection in water supplies is not well served by existing lab techniques. We develop a new multi-modal platform comprising functionalized quantum dots of two-dimensional materials (2D-QDs) for the sensing of four highly-toxic heavy metal pollutants (arsenic, cadmium, lead and mercury). The zero-dimensional […]
March 11, 2019
Implementing High-fidelity Quantum Gates in Multi-level Trapped Ions
Summary The scalability of quantum processors is limited by current error rates for single-qubit gates. By encoding more than a single bit of information within a single ion, multi-level “qudits” offer a promising method of increasing the information density within a quantum processor, and therefore minimizing the number of gates and associated error rates. […]
July 30, 2018